Time has two aspects. For civil and some scientific purposes, we want to know the time of day so that we can order events in sequence. In much scientific work, we want to know how long an event lasts. Thus, any time standard must be able to answer two questions: “When did it happen?” and “What is its duration?” Table 1-4 shows some time intervals.
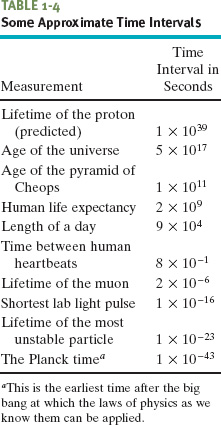
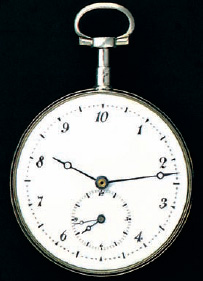
Fig. 1-1 When the metric system was proposed in 1792, the hour was redefined to provide a 10-hour day. The idea did not catch on. The maker of this 10-hour watch wisely provided a small dial that kept conventional 12-hour time. Do the two dials indicate the same time?
Any phenomenon that repeats itself is a possible time standard. Earth’s rotation, which determines the length of the day, has been used in this way for centuries; Fig. 1-1 shows one novel example of a watch based on that rotation. A quartz clock, in which a quartz ring is made to vibrate continuously, can be calibrated against Earth’s rotation via astronomical observations and used to measure time intervals in the laboratory. However, the calibration cannot be carried out with the accuracy called for by modern scientific and engineering technology.
To meet the need for a better time standard, atomic clocks have been developed. An atomic clock at the National Institute of Standards and Technology (NIST) in Boulder, Colorado, is the standard for Coordinated Universal Time (UTC) in the United States. Its time signals are available by shortwave radio (stations WWV and WWVH) and by telephone (303-499-7111). Time signals (and related information) are also available from the United States Naval Observatory at Web site http://tycho.usno.navy.mil/time.html. (To set a clock extremely accurately at your particular location, you would have to account for the travel time required for these signals to reach you.)
Figure 1-2 shows variations in the length of one day on Earth over a 4-year period, as determined by comparison with a cesium (atomic) clock. Because the variation displayed by Fig. 1-2 is seasonal and repetitious, we suspect the rotating Earth when there is a difference between Earth and atom as timekeepers. The variation is due to tidal effects caused by the Moon and to large-scale winds.
The 13th General Conference on Weights and Measures in 1967 adopted a standard second based on the cesium clock:
One second is the time taken by 9 192 631 770 oscillations of the light (of a specified wavelength) emitted by a cesium-133 atom.
Atomic clocks are so consistent that, in principle, two cesium clocks would have to run for 6000 years before their readings would differ by more than 1 s. Even such accuracy pales in comparison with that of clocks currently being developed; their precision may be 1 part in 1018—that is, 1 s in 1 × 1018 s (which is about 3 × 1010 y).
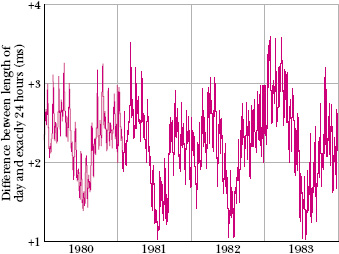
Fig. 1-2 Variations in the length of the day over a 4-year period. Note that the entire vertical scale amounts to only 3 ms (= 0.003 s).
The ghostly clouds in this chapter’s opening photograph first appeared following the huge 1883 volcanic explosion of Krakatoa Island (near Java in the southeast Pacific). The explosion was so violent that it hurled dust to the mesosphere, a cool portion of the atmosphere located well above the stratosphere. There water collected and froze on the dust to form the particles that made the first of these clouds. Since then, the clouds have frequently reappeared not because of volcanic explosions but because of the increased production of methane by industries, rice paddies, landfills, and livestock flatulence. The methane works its way into the upper atmosphere and undergoes chemical changes, resulting in an increase of water molecules and the bits of ice needed for the formation of the mesospheric clouds, as they are now called.
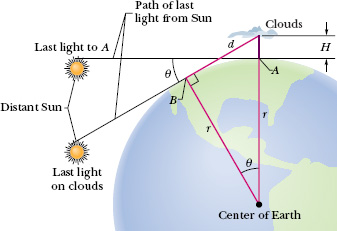
Fig. 1-3 The last sunlight reaching an observer at point A occurs at sunset. The last sunlight reaching clouds at height H above the observer occurs later, after the Sun appears to rotate through an angle θ. Height H and angle θ are exaggerated for clarity.
The mesospheric clouds are visible after sunset because they are in the upper portion of the atmosphere that is still illuminated by sunlight. They are not visible earlier in the day in spite of their illumination because the lower atmosphere is then too brightly lit for them to be distinguished. If the clouds are spotted overhead 38 min after sunset and then quickly dim, what is their altitude H?
Solution: Figure 1-3 shows the situation for the observer, who is at point A on Earth’s surface, below the mesospheric clouds at altitude H. A Key Idea is that at sunset, the last sunlight reaching the observer follows a path that is tangent to Earth’s surface at point A. A second Key Idea is that the last sunlight reaching the mesospheric clouds above the observer follows a path that is tangent to Earth’s surface at point B. This occurs at time t = 38 min after sunset.
From Fig. 1-3, the angle between these two paths is θ, the angle through which the Sun appears to move about Earth during the 38 min. During a full day, which is approximately 24 h, the Sun appears to move through an angle of 360°. Thus, in time t = 38 min, the Sun appears to move through an angle

From Fig. 1-3, this angle θ is also the angle between the Earth radii r to the two tangent points A and B. The figure shows a right triangle: one leg is r and the hypotenuse is r + H. Using the definition of the cosine function from trigonometry, we can write

From Appendix C, the (mean) radius of Earth is r = 6.37 × 106 m. Substituting this and θ = 9.50° into Eq. 1-7, we have

which gives us

The more frequent occurrence of the clouds in recent decades indicates that methane production on Earth’s surface is changing even the mesosphere.
Leave a Reply